Ecosystems beyond Earth
View Sequence overviewStudents will:
- examine how to observe a plant.
- identify independent, dependent and controlled variables.
- plan a reproducible experiment to test the relationship between coloured light and plant growth.
Students will represent their understanding as they:
- draw a diagram of their observations of a plant.
- plan and conduct a safe, reproducible experiment to test the relationship between coloured light and plant growth.
- select and construct appropriate representations to organise data and information.
In this lesson, assessment is formative.
Feedback might focus on:
- representations of flows of energy in an ecosystem.
- planing and conducting safe, reproducible investigations to test relationships.
- selecting and constructing appropriate representations to organise data and information.
Potential summative assessment
Students working at the achievement standard should:
- represent flows of energy in ecosystems.
- plan and conduct safe, reproducible investigations to test relationships.
- use equipment to generate and record data with precision.
Refer to the Australian Curriculum content links on the Our design decisions tab for further information.
Whole class
Ecosystems beyond Earth Resource PowerPoint
Sticky notes
Whiteboard to write on (with associated markers)
Display plant e.g. lettuce seedling (also used in Lesson 3)
Each group
8 long skewers
Masking or sticky tape
Coloured cellophane (red, blue, and green)
Well-lit area
Water
Seedling lettuce plants or duckweed plants (minimum 2/group)
Soil or water for the plants to grow in
Small containers to hold the seedlings (i.e. egg cartons)
Dish to contain water under the plants
Each student
Individual notebook
Testing plant growth Resource sheet
Lesson
The Inquire phase allows students to cycle progressively and with increasing complexity through the key science ideas related to the core concepts. Each Inquire cycle is divided into three teaching and learning routines that allow students to systematically build their knowledge and skills in science and incorporate this into their current understanding of the world.
When designing a teaching sequence, it is important to consider the knowledge and skills that students will need in the final Act phase. Consider what the students already know and identify the steps that need to be taken to reach the level required. How could you facilitate students’ understanding at each step? What investigations could be designed to build the skills at each step?
Read more about using the LIA FrameworkRe-orient
Recall the previous lesson, focusing on what we know about the requirements of sending astronauts to the Moon or Mars.
Watch the video Off limits: Space food - Find out what astronauts eat in space (3:36).
Discuss the importance of food to supply us with the energy that we need to move, repair tissue/muscles, and grow.
The Inquire phase allows students to cycle progressively and with increasing complexity through the key science ideas related to the core concepts. Each Inquire cycle is divided into three teaching and learning routines that allow students to systematically build their knowledge and skills in science and incorporate this into their current understanding of the world.
When designing a teaching sequence, it is important to consider the knowledge and skills that students will need in the final Act phase. Consider what the students already know and identify the steps that need to be taken to reach the level required. How could you facilitate students’ understanding at each step? What investigations could be designed to build the skills at each step?
Read more about using the LIA FrameworkIdentifying and constructing questions is the creative driver of the inquiry process. It allows students to explore what they know and how they know it. During the Inquire phase of the LIA Framework, the Question routine allows for past activities to be reviewed and to set the scene for the investigation that students will undertake. The use of effective questioning techniques can influence students’ view and interpretation of upcoming content, open them to exploration and link to their current interests and science capital.
When designing a teaching sequence, it is important to spend some time considering the mindset of students at the start of each Inquire phase. What do you want students to be thinking about, what do they already know and what is the best way for them to approach the task? What might tap into their curiosity?
Read more about using the LIA FrameworkWhat do plants need?
Discuss the challenges of supplying food to astronauts when they are further away from Earth (such as on the Moon and Mars).
(Slide 9) Recall food chains all start with a plant and that plants need sunlight.
- What foods do astronauts need?
- A variety of different foods and fibre so they stay healthy and fit for space.
- Where are we/humans located in a food chain?
- We are omnivores that eat plants and other animals. Therefore, we are second or third (trophic level) on the food chain.
- What is at the start of all food chains?
- Plants.
- How do plants get their energy?
- Sunlight.
- Does the International Space Station (ISS) have natural light?
- No. It uses artificial light.
Pose the question: Does it matter what colour of light the plants are exposed to?
Explain that students will be testing this during the teaching sequence.
Pose the question: How do we know if a plant is growing well under coloured light?
Nutrition in space
Long-distance space travel requires planning.
On the International Space Station (ISS), astronauts have had access to a safe food system with resupply missions bringing fresh food and psychological benefits. However, upcoming lunar missions may have more constrained food systems due to limited resources, with potential issues arising from a diet of cold, shelf-stable foods. A test of meal replacement bars for lunar missions showed reduced food intake and a decrease in astronaut moods. A mission to Mars will face even greater challenges, with crews spending up to 9 months travelling to Mars, living on the planet for 18 months, and returning to Earth, without the possibility of resupply.
Developing a food system for such long-duration missions means we need to consider:
- Safety: Minimising food-related health risks is crucial, including ensuring that food doesn’t introduce harmful microorganisms or waste streams.
- Stability: Mars missions will need food to last for up to 5 years, but the current technology doesn’t allow for this.
- Palatability: Even if food is safe and nutritious, moral and maintaining weight rely on a pleasing taste.
- Nutrition: Past expeditions failed due to a lack of essential nutrients.
- Resource minimisation: Every food system must be designed to reduce mass, volume, water, and power, and to manage waste efficiently.
- Variety: A mix of food types and flavours is necessary to avoid menu fatigue and ensure astronauts are motivated to eat a balanced diet.
- Reliability: The food system must be fail-safe in space conditions (e.g., microgravity, radiation), as any failure could endanger the mission.
- Usability: Food preparation must be quick and easy for astronauts.
- Space-ready appliances: Cooking in space poses unique challenges, requiring new appliances and systems to ensure food preparation is safe and feasible.
On the International Space Station (ISS), astronauts have had access to a safe food system with resupply missions bringing fresh food and psychological benefits. However, upcoming lunar missions may have more constrained food systems due to limited resources, with potential issues arising from a diet of cold, shelf-stable foods. A test of meal replacement bars for lunar missions showed reduced food intake and a decrease in astronaut moods. A mission to Mars will face even greater challenges, with crews spending up to 9 months travelling to Mars, living on the planet for 18 months, and returning to Earth, without the possibility of resupply.
Developing a food system for such long-duration missions means we need to consider:
- Safety: Minimising food-related health risks is crucial, including ensuring that food doesn’t introduce harmful microorganisms or waste streams.
- Stability: Mars missions will need food to last for up to 5 years, but the current technology doesn’t allow for this.
- Palatability: Even if food is safe and nutritious, moral and maintaining weight rely on a pleasing taste.
- Nutrition: Past expeditions failed due to a lack of essential nutrients.
- Resource minimisation: Every food system must be designed to reduce mass, volume, water, and power, and to manage waste efficiently.
- Variety: A mix of food types and flavours is necessary to avoid menu fatigue and ensure astronauts are motivated to eat a balanced diet.
- Reliability: The food system must be fail-safe in space conditions (e.g., microgravity, radiation), as any failure could endanger the mission.
- Usability: Food preparation must be quick and easy for astronauts.
- Space-ready appliances: Cooking in space poses unique challenges, requiring new appliances and systems to ensure food preparation is safe and feasible.
The Inquire phase allows students to cycle progressively and with increasing complexity through the key science ideas related to the core concepts. Each Inquire cycle is divided into three teaching and learning routines that allow students to systematically build their knowledge and skills in science and incorporate this into their current understanding of the world.
When designing a teaching sequence, it is important to consider the knowledge and skills that students will need in the final Act phase. Consider what the students already know and identify the steps that need to be taken to reach the level required. How could you facilitate students’ understanding at each step? What investigations could be designed to build the skills at each step?
Read more about using the LIA FrameworkThe Investigate routine provides students with an opportunity to explore the key ideas of science, to plan and conduct an investigation, and to gather and record data. The investigations are designed to systematically develop content knowledge and skills through increasingly complex processes of structured inquiry, guided inquiry and open inquiry approaches. Students are encouraged to process data to identify trends and patterns and link them to the real-world context of the teaching sequence.
When designing a teaching sequence, consider the diagnostic assessment (Launch phase) that identified the alternative conceptions that students held. Are there activities that challenge these ideas and provide openings for discussion? What content knowledge and skills do students need to be able to complete the final (Act phase) task? How could you systematically build these through the investigation routines? Are there opportunities to build students’ understanding and skills in the science inquiry processes through the successive investigations?
Read more about using the LIA FrameworkPlant growth experiments
Display a plant for students to observe.
✎ STUDENT NOTES: Draw a diagram of the plant.
Discuss the importance of drawing what they see rather than what they expect to see.
- How many leaves does your plant have?
- What shape are the leaves?
- Do they have smooth edges or bumps/serrated edges?
- Is there a vein/xylem down the middle?
- Are there smaller veins coming off a central vein?
- Is the leaf one colour or darker in some places?
- How are the leaves attached?
- What labels or measurements does you picture need?
(Slide 10) Brainstorm all the ways a plant can be measured. Options include the number/colour/length/height/width of leaves and number/length/depth/width of roots.
✎ STUDENT NOTES: Record ideas.
(Slide 11) Brainstorm all the things/variables that may change the way a plant will grow. Record each variable on a sticky-note and display on a board or window. Make sure ‘coloured light’ is included.
Discuss the meaning of an independent variable and move the sticky-note with ‘coloured light’ to one side. Label it ‘independent variable’.
Discuss what would be the most reliable way to measure plant growth (dependent variable).
Students are likely to suggest using plant height as a way to measure plant growth. However, when lettuce leaves grow in green light, they will initially grow taller in an attempt to reach light with greater energy, before withering. For this reason height is not a good choice of dependent variable.
Optional: Different student groups may decide to measure different dependent variables.
Group the remaining variables as ‘controlled variables’. Discuss how it is easy to make assumptions about the controlled variables (e.g. assume that the temperature near windows is the same as the temperature in the centre of the room).
- What does the word ‘controlled’ mean?
- To make sure that each plant is in the same conditions except for the one change that we make (independent variable).
- Why is it important to keep the conditions between all the plants the same (except for the independent variable)?
- So we know that the variable that we select is the one that caused the change.
- How can we control the variables we have identified?
- How could we measure these variables to ensure that one plant does not have different conditions from other plants?
✎STUDENT NOTES: Record the different variables and how they will be controlled in the Testing plant growth Resource sheet (Slide 12). Plan your experiment and consider any possible risks involved.
Allow students time to set up their experiments. Consider:
- using the skewers and masking tape to make a pyramid scaffold for the cellophane. If this is done carefully, the pyramids can be stored and reused in the following years).
- using double layers of cellophane (especially the green) provides for more thorough filtering of the light reaching the leaves of the plants.
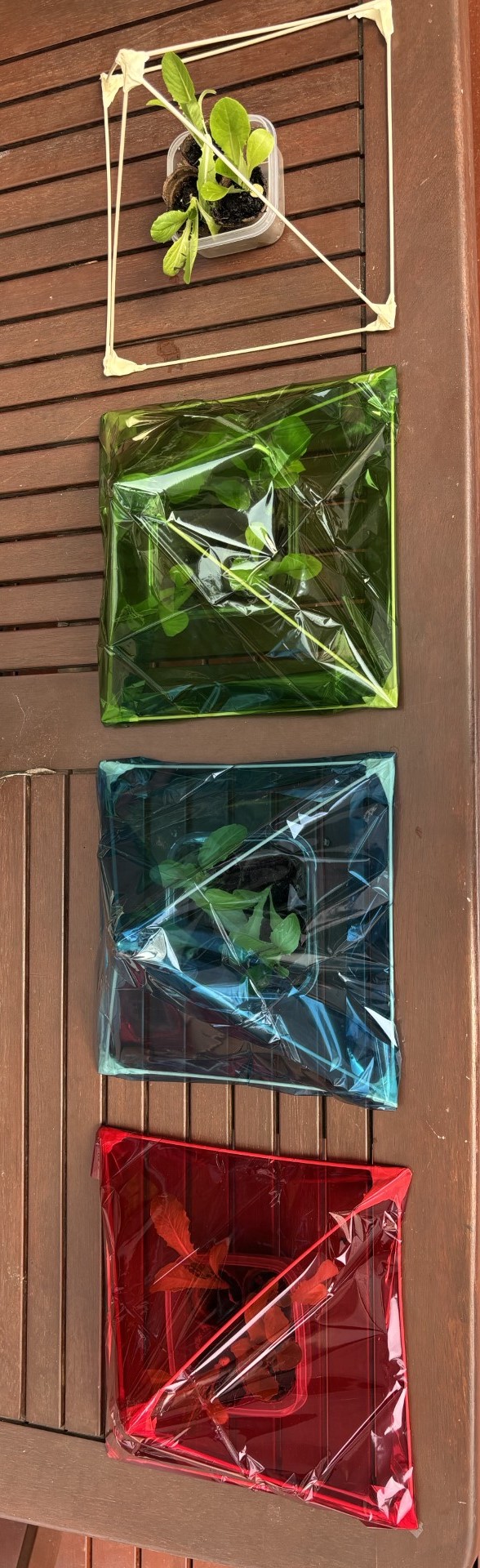
Plant structure
What do you know about plant structure?
Plants have a hierarchical structure that is organised into different levels: cells, tissues, organs, and systems. These components work together to support the plant’s growth, reproduction, and survival. As students will learn in Year 8, plants have the following organs.
- Roots: Anchor the plant to the soil, absorb water and nutrients, and store food.
- Stems: Support the plant, transport nutrients (phloem) and water (xylem) between the roots and leaves, and can also store food.
- Leaves: The primary site for photosynthesis, where light energy is converted into chemical energy (glucose).
- Flowers (in flowering plants): Reproductive organs that enable sexual reproduction through pollination. The parts include sepals, petals, stamens (male reproductive organs), and pistils (female reproductive organs).
- Fruits: Mature ovaries containing seeds, which protect and disperse the plant’s offspring.
Each organ has a specific function that supports the overall life cycle of the plant, from growth and energy production to reproduction and seed dispersal.
Plants have a hierarchical structure that is organised into different levels: cells, tissues, organs, and systems. These components work together to support the plant’s growth, reproduction, and survival. As students will learn in Year 8, plants have the following organs.
- Roots: Anchor the plant to the soil, absorb water and nutrients, and store food.
- Stems: Support the plant, transport nutrients (phloem) and water (xylem) between the roots and leaves, and can also store food.
- Leaves: The primary site for photosynthesis, where light energy is converted into chemical energy (glucose).
- Flowers (in flowering plants): Reproductive organs that enable sexual reproduction through pollination. The parts include sepals, petals, stamens (male reproductive organs), and pistils (female reproductive organs).
- Fruits: Mature ovaries containing seeds, which protect and disperse the plant’s offspring.
Each organ has a specific function that supports the overall life cycle of the plant, from growth and energy production to reproduction and seed dispersal.
Photosynthesis and coloured light
Which colour of light is most effective for photosynthesis?
Photosynthesis is the process by which plants use carbon dioxide and water to convert light energy into chemical energy (glucose) and oxygen. This process occurs through the main pigment chlorophyll and minor pigments including carotenoids and anthocyanines.
The efficiency of photosynthesis is influenced by the type (or colour) of light, as different wavelengths of light affect the plant’s ability to produce chemical energy in the form of glucose.
Blue and red light are most effective for photosynthesis because chlorophyll absorbs these wavelengths efficiently.
Green light is not absorbed much by chlorophyll, so it has a lesser direct effect on photosynthesis but still contributes in small amounts due the presence of pigments other than chlorophyll. The reflection of green light is the reason most plants appear green.
The quality (colour) and intensity of light affect both the rate of photosynthesis and overall plant growth, with blue light promoting compact growth and red light encouraging flowering and fruiting.
In controlled environments, such as indoor farming or research labs, artificial lights such as LEDs are used to optimise photosynthesis. These lights are tuned to emit specific wavelengths (usually blue and red) to maximise plant growth and productivity.
Photosynthesis is the process by which plants use carbon dioxide and water to convert light energy into chemical energy (glucose) and oxygen. This process occurs through the main pigment chlorophyll and minor pigments including carotenoids and anthocyanines.
The efficiency of photosynthesis is influenced by the type (or colour) of light, as different wavelengths of light affect the plant’s ability to produce chemical energy in the form of glucose.
Blue and red light are most effective for photosynthesis because chlorophyll absorbs these wavelengths efficiently.
Green light is not absorbed much by chlorophyll, so it has a lesser direct effect on photosynthesis but still contributes in small amounts due the presence of pigments other than chlorophyll. The reflection of green light is the reason most plants appear green.
The quality (colour) and intensity of light affect both the rate of photosynthesis and overall plant growth, with blue light promoting compact growth and red light encouraging flowering and fruiting.
In controlled environments, such as indoor farming or research labs, artificial lights such as LEDs are used to optimise photosynthesis. These lights are tuned to emit specific wavelengths (usually blue and red) to maximise plant growth and productivity.
The Inquire phase allows students to cycle progressively and with increasing complexity through the key science ideas related to the core concepts. Each Inquire cycle is divided into three teaching and learning routines that allow students to systematically build their knowledge and skills in science and incorporate this into their current understanding of the world.
When designing a teaching sequence, it is important to consider the knowledge and skills that students will need in the final Act phase. Consider what the students already know and identify the steps that need to be taken to reach the level required. How could you facilitate students’ understanding at each step? What investigations could be designed to build the skills at each step?
Read more about using the LIA FrameworkFollowing an investigation, the Integrate routine provides time and space for data to be evaluated and insights to be synthesized. It reveals new insights, consolidates and refines representations, generalises context and broadens students’ perspectives. It allows student thinking to become visible and opens formative feedback opportunities. It may also lead to further questions being asked, allowing the Inquire phase to start again.
When designing a teaching sequence, consider the diagnostic assessment that was undertaken during the Launch phase. Consider if alternative conceptions could be used as a jumping off point to discussions. How could students represent their learning in a way that would support formative feedback opportunities? Could small summative assessment occur at different stages in the teaching sequence?
Read more about using the LIA FrameworkFlow of energy in space
Discuss why it is important to make initial measurements of the plants. Confirm with the students what dependent variable(s) they will be measuring in a reproducible way.
- What type of measurements or observations will you make?
- Will your data be quantitative (numerical) or qualitative (descriptions)?
- How will you record your data?
- What headings might you need in your table?
- What units will you use when measuring?
- If all leaves are different lengths to start with, how could you show any change? How could you show this in your table?
✎ STUDENT NOTES: Draw up a table for the initial measurements of the plants.
(Slide 13) Discuss how energy cannot be created or destroyed, but it could be difficult to reuse or lost to the system.
✎ STUDENT NOTES: Record the pathway/transfer of energy on the ISS.
Depending on students’ prior knowledge, they may omit the type of energy (text in red).

- Where do the plants get the energy to grow?
- Where does the light energy come from?
- What about the ISS, where does its energy come from?
- The ISS has solar panels to capture sunlight. This light energy is transformed into electrical energy for the LED lights, which convert it back to light energy for the plants.
- If the astronauts get their energy from eating the plants, where does their energy go?
- The energy is transferred to movement/kinetic energy, and thermal/heat energy. This helps to heat the air in the ISS.
- Where does the heat energy go?
- The ISS has a cooling system to remove the thermal/heat energy from the inside of the ISS through radiation.
Optional: Watch the video How do you grow plants in space? | BBC News (4:19).
Reflect on the lesson
You might:
- watch the video Bean Time-Lapse - 25 days | Soil cross section (3:09) and describe the growth of the seed at Day 1, 4, 7, 9, and 12.
- re-examine the intended learning goals for the lesson and consider how they were achieved.
- discuss how students were thinking and working like scientists during the lesson. Focus on the planning of reproducible experiments.
Energy in an ecosystem
Your students may hold alternative conceptions about the movement of energy through systems.
One of the key concepts in this teaching sequence is examining how energy moves in a system. In this instance, the system is the International Space Station. Common alternative conceptions held by students include:
- energy is recycled in an ecosystem.
- producers use all the sunlight that reaches them.
- all of the energy in a trophic level is passed on to the next level.
- a larger animal always has more energy.
- energy flow is the same in all ecosystems.
- producers don’t lose energy.
One of the key concepts in this teaching sequence is examining how energy moves in a system. In this instance, the system is the International Space Station. Common alternative conceptions held by students include:
- energy is recycled in an ecosystem.
- producers use all the sunlight that reaches them.
- all of the energy in a trophic level is passed on to the next level.
- a larger animal always has more energy.
- energy flow is the same in all ecosystems.
- producers don’t lose energy.