Forces change the way things move. Forces can make stationary objects move and moving objects speed up, slow down or stop. They can change the direction an object is moving and can even change its shape. It is not possible to ‘see’ a force—it is only possible to feel or observe its effects.
Force has two aspects: magnitude and direction. The magnitude of the force refers to the size, or amount, of force exerted, for example, a strong or a weak kick (push force) to a soccer ball.
Force-arrow diagrams are used to represent the direction and the size of forces acting in a particular situation. The length of a force-arrow represents magnitude and the direction of the arrow shows the direction in which the force is acting. When drawing force-arrow diagrams, longer arrows in the direction of the force are used to represent a larger force while a shorter arrow in the direction of the force is used to represent a smaller force. Asking students to label the force-arrows will assist with their representations and explanations of forces and motion in the early years.
At more senior levels the arrows represent vectors where the direction of the arrow represents the direction of the vector, and the length of the arrow represents the magnitude of the vector measured in newtons. The method of adding these vectors, called the head-to-tail method, is the way vectors must be added. More complex methods involving trigonometry are an application of this theoretical idea.
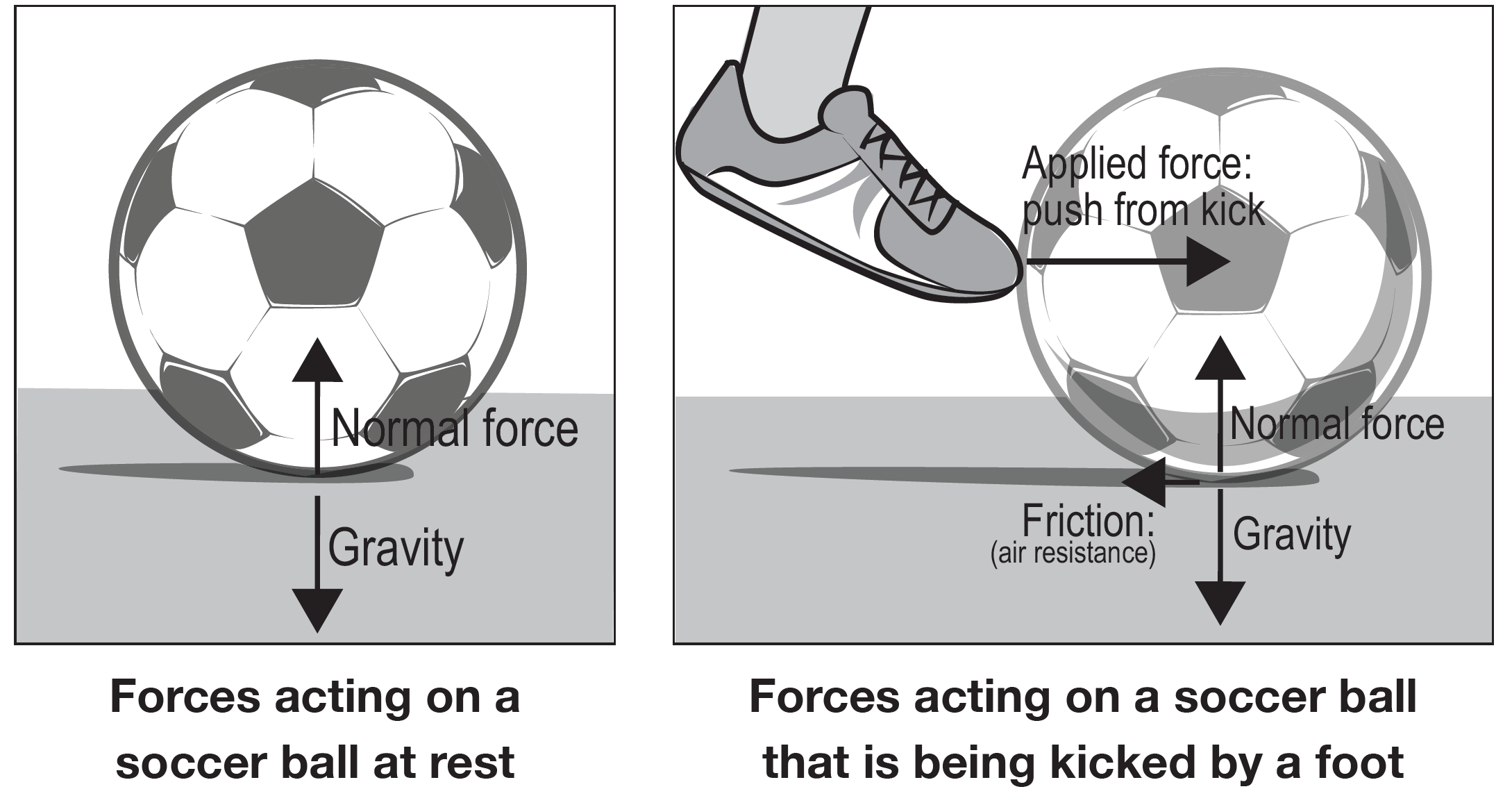
Note: The diagrams above are not force-arrow diagrams in the strictest sense, as scientists would portray all forces acting on an object’s centre of mass. It is also not what you would expect students to be able to draw. A ‘normal force’ is the force perpendicular to the two surfaces in contact and is generally a result of resisting the pull of gravity.
Forces come in pairs—one force is an action while the second force is a reaction. For example, our standing body is pulled down to the ground and the ground pushes back against our feet. We are able to be stationary because even though there are two forces acting on us, these forces are of equal magnitude in opposite directions. The forces are therefore said to be balanced.
A stationary object has forces acting on it, but it is stationary because those forces are balanced. For example, a soccer ball at rest (see diagram above left), remains stationary because there are two forces of equal magnitude acting on it: the pull from gravity and the opposing push from surfaces (normal force).
A free-body diagram shows all the forces acting on a single object. The term ‘free body diagram’ is often not included in the teaching of forces at this level even though students are often required to place multiple force arrows on a diagram to represent multiple forces acting. There is no great conceptual leap in identifying that there is a scientific term for such diagrams. An important feature of free-body diagrams is that the tail of the force arrow is placed as close as possible to where the force acts. Students will often place the arrowhead in this location to indicate where the object is being pushed.
The result should be a diagram with multiple force arrows showing the different forces acting on one object. The free body diagram is a fundamental instrument used by physicists and engineers to describe the force interactions in any situation. It can be a very simple representation, as shown in this diagram:
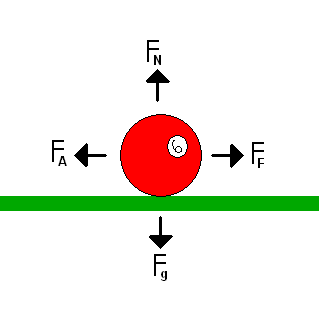
Some forces are worth a particular mention. $F_A$ is the force that is being applied (for example a push or pull). $F_F$ is the frictional force. $F_g$ is the gravitational force that is pulling the object towards the centre of the Earth. A normal force ($F_N$) is one acting from a surface onto an object resting on it. The size of the normal force will be equal in size to the force of the object pressing on it (possibly its weight if it is lying horizontally) and they are always perpendicular to the surface in question. The force of friction is a horizontal force exerted by any surface that is experiencing a force due to some other surface applying a horizontal force to it. If there is no horizontal component to any force between the two surfaces then there is no force of friction occurring. For example, if a book is simply lying on a table, there is no friction. If someone attempts to slide the book across the table then there will be a frictional force occurring, whether or not the book moves.
Friction is sometimes referred to as a force that opposes motion, but this is not strictly correct. If an object is changing direction the force of friction will relate to the direction the object is accelerating rather than the direction of its current motion. An object at rest may be experiencing many forces including static friction. The gravitational force acts on all objects and is generally shown acting either from the centre or the bottom of the object directly downwards.
Newton’s laws
Newton's First Law of Motion, also known as the Law of Inertia, states that "An object will remain at rest, or move in a straight line with constant speed unless acted upon by an external force."
This law explains the behaviour of objects in terms of their motion (or lack thereof) and the role of forces in changing that motion. In fact, the first law is simply a consequence of the second law, which states that $a = \frac{F}{m}$. If $F = 0$, then it follows that $a = 0$, which is simply what the first law states.
Inertia is the tendency of objects to resist changes in their state of motion. It’s the cause of a jolt when a car suddenly starts or stops; your body wants to stay in its current state (at rest or in motion) because of inertia. The greater the mass of an object, the greater its inertia. That means heavier objects are harder to start moving and harder to stop once they are moving.
If no external force acts on an object, it will either remain stationary (if it’s at rest) or continue moving at a constant speed in a straight line (if it’s already moving). For example, if you slide a hockey puck on ice, it will keep sliding in a straight line at a constant speed unless forces like friction or a player’s stick act on it.
Newton’s Second Law of Motion, $F=ma$, outlines that forces of different magnitudes have different effects on different objects. For example, a big push will make a swing accelerate quicker. The effect of a force on an object also depends on the object’s mass, which is the amount of matter in the object. For example, when we run, we have more kinetic energy than when we walk, hence a bigger force is needed to make us stop. The same is true for cars; it is easier to stop a car when it is moving slowly than when it is moving fast. This is why slower speed limits are in place around areas where there might be lots of people, such as schools, hospitals, and shopping centres. Objects with more mass require a larger initial force to move at the same speed as objects with less mass. Similarly, a more massive object will require a larger force to stop it moving than an object with less mass travelling at the same speed. For example, at a given speed, stopping a bicycle requires less force than stopping a car, and both require less force than stopping a truck.
Newton's Third Law of Motion states: "For every action, there is an equal and opposite reaction." This law explains how forces always come in pairs. When one object applies a force on another object, the second object applies a force of equal magnitude but in the opposite direction on the first object.
This terminology implies that one of the forces is the initiator of the interaction and that the other is a response. The correct idea is that every force represents an interaction (specifically a push or a pull) between two objects. There is no such thing as a single force.
A very common confusion in this topic is between force pairs and balanced forces. Force pairs are fundamental to the nature of a force. The two forces in a force pair are acting on two different objects that are interacting. They always have the same magnitude but opposite directions. Balanced forces represent more than one force acting on the same object in such a way that they cancel each other out. In mathematical terms, the force vectors (or simply the forces) add up to zero.
Multiple forces acting on one object may or may not add up to zero. If they do then there is no resultant change in the object’s motion.
Friction
When an object is moving over a surface, the contact between it and the surface creates friction. Friction occurs between all types of materials whether they are solids, liquids, or gases. Friction is caused by the interaction between surfaces causing them to ‘stick’ together and oppose the movement between them.
To make something move, we need to apply enough force (transfer enough energy to the object) to overcome the force of friction opposing that acceleration of movement. Moving objects slow down and stop because the force of friction acts against the movement (transforms the movement energy to heat, and sometimes sound energy) and slows the object to a stop. When depicting friction on force-arrow diagrams the friction force-arrow is drawn in the direction opposing direction of movement or acceleration of movement and will always be smaller or equal to the push/pull force.
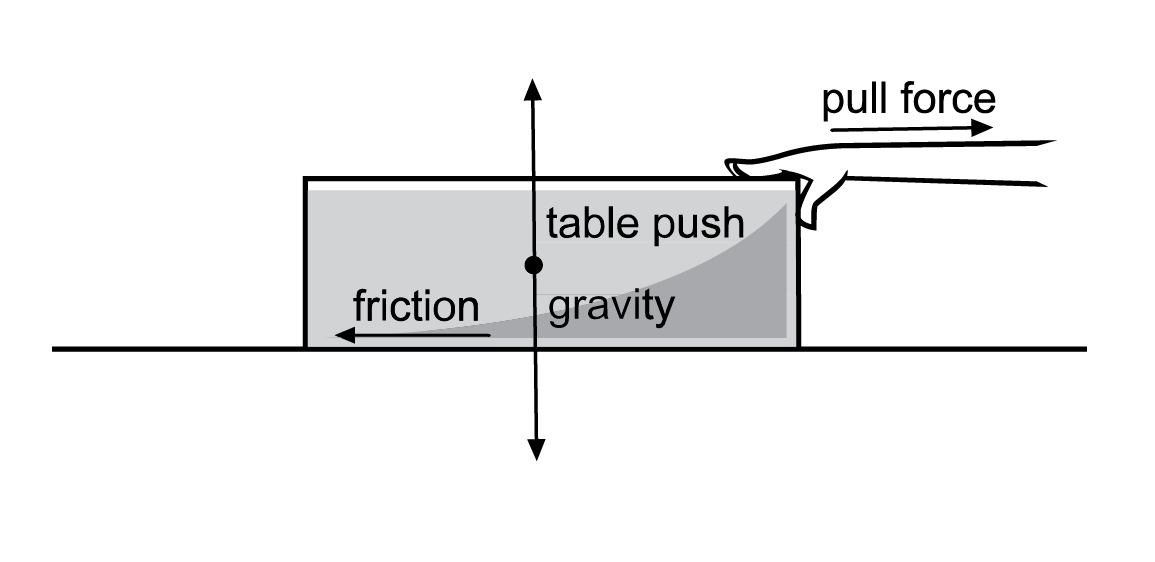
Gravitational force
Every object exerts a gravitational force on other objects but it can be hard to detect unless at least one of the objects has a large mass. ‘Mass’ is a measure of the amount of matter an object has while ‘weight’ is the measure of gravitational pull that acts on an object. The weight of an object is related to the mass of the object and the magnitude of the gravitational force acting on the object.
The weight of an object will change if the gravitational force acting on it changes, but its mass will not. For example, the Moon is not as massive as the Earth so its gravitational force is not as strong. Because of this, objects will not be attracted as strongly to the centre of the Moon as they would be to the centre of the Earth, and their weight on the Moon is less than their weight on Earth.
The Earth has such a large mass that the gravitational attraction between it and most things is very noticeable; when we jump into the air, the Earth’s gravitational force pulls us back towards the Earth’s centre very quickly. We can also feel the pull of the Earth’s gravity when we try to lift things; the more mass something has, the greater the pull of gravity and the greater the lifting force we need to use. The pull of gravity acts on an object regardless of whether or not the object is moving. It does not require the object to be surrounded by air or water or anything else and can therefore act in the vacuum of space.
Magnets
A magnet is a material that generates a magnetic field. Magnets only attract materials composed of iron, nickel and cobalt. These materials are described as being ‘magnetic’. Steel is attracted to magnets as it is mostly made of iron. Most other materials such as wood, plastic, aluminium, silver and copper are not attracted to magnets and are called ‘non-magnetic’.
A magnetic field is the region around a magnet in which the magnet exerts force. Many small pieces of iron, called iron filings, are used to show the magnetic field around a magnet. The iron filings form a pattern of lines called magnetic field lines. The arrangement of the magnetic field lines depends on the shape of the magnet, but the lines always extend from one pole to the other pole. The force is weaker farther away from the magnet.
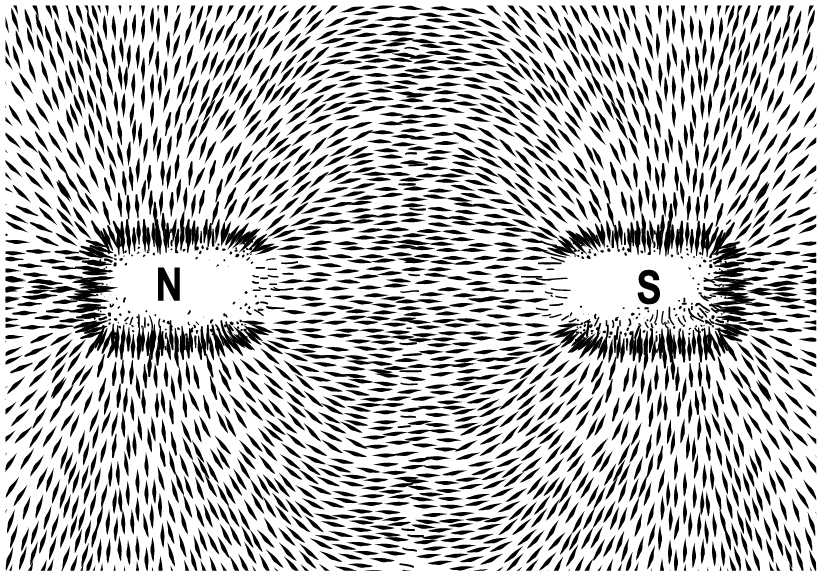
Linking force and energy
When forces are applied to an object, energy is transferred or transformed. When a football player kicks a ball, movement energy in his foot is transferred to the ball. When hands are rubbed together, friction transforms movement energy into heat energy. When you turn on a switch, electrical energy from the power station is transformed into light energy in your room.
Simple machines
Simple machines usually achieve one, or both, of two things. They change the direction of a force and/or multiply force. The multiplication of force is called the mechanical advantage.
Energy is a property of something that indicates its potential for change (ability to do work). Energy is always conserved, but it can be transferred or transformed.
Energy can appear abstract to students, but they can often detect it through the effect it has on their body: they can see patterns of light, and they can feel the warmth created by heat energy, though they cannot see or feel magnetic energy. Energy from the Sun drives the growth of plants and the development of rainstorms, while energy from chemical reactions gives life to animals and is also important to modern industry.
Some important characteristics of energy include:
- Energy exists in different forms, such as light, sound, heat, electricity and movement.
- Energy can be transformed (changed) from one form to another, for example, kicking a ball transforms chemical energy in our bodies to movement energy in the ball.
- Energy can be transferred from one location to another, for example, electrical energy moves along wires from a power station to our houses.
- Energy can be changed into other forms, but it cannot be created or destroyed.
- Energy can be stored in many ways. Batteries and fossil fuels are stores of chemical energy.
- Energy is the capacity to do work.
- Energy transfers involve change.
- Energy looks different in different situations—it can be transferred from one object to another.
- Electricity is a major way of transferring energy to our cities and homes.
- Heat/thermal energy is the energy that flows from hot to cold objects.
- Temperature is a measure of how hot or cold an object is, as measured by the thermometer.
- Energy transfer through different mediums can be explained using wave and particle models.
- Heat will flow from a hot object to a colder object until they are at the same temperature (equilibrium).
- Heat can be transferred through conduction, convection or radiation.
- It takes a lot of energy to heat or boil water and to melt ice.
Identifying energy as different energy forms may imply that energy is a material substance. This can lead to confusion for many students. The key aspect to consider is when something changes, energy is involved.
Sankey diagrams
Sankey diagrams summarise all the energy transfers underway in a process. The thickness of each line is proportional to the amount of energy involved. Sankey diagrams are named after Irish Captain Matthew Henry Phineas Riall Sankey, who used this type of diagram in 1898 in a publication on the energy efficiency of a steam engine.
This Sankey diagram for an electric lamp shows that most of the electrical energy is transferred as heat rather than light.
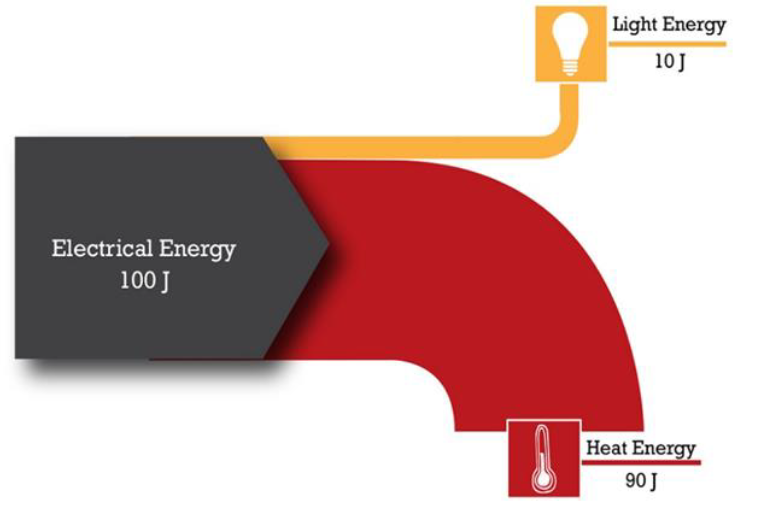
Kinetic energy and gravitational potential energy example
Most teachers start by throwing a ball in the air and describing the kinetic energy (KE) of the ball being transformed into gravitational potential energy (GPE). KE is initially provided to a ball while it is still in contact with the throwing hand. Once the ball leaves the hand there is no input of energy. As the ball rises the KE is transformed to GPE. This GPE is effectively stored in the height of the ball. (Strictly it is stored in the greater distance between the ball and the centre of the Earth.)
At the top of the flight, the ball stops momentarily (a difficult concept) at which point it has zero KE and maximum GPE. As the ball falls and loses height, the GPE is transformed into kinetic energy again.
Together kinetic and gravitational potential energies are referred to as mechanical energy.
Further information will be provided in the future.
Alternative conception | Accepted conception |
---|---|
Heat is an entity or a measure of hotness. | Heat is the flow of energy from a warm object to a cooler one. |
Heat only travels upwards. | Heat can travel upwards, but it can also travel in other directions through conductions and radiation. |
Heat and temperature are the same. | Heat is the transfer of energy between two objects that are at different temperatures. Temperature is a measure of the kinetic energy of an object. |
Heat is an entity or a measure of hotness. | Heat is the flow of energy from a warm object to a cooler one. |
Energy is used up. | Energy may be transformed or transferred but it is always conserved. |
Energy is a ‘thing’. | Materials and objects can have energy transferred to them. |
Energy and force are interchangeable. | A force is an external effect that can cause an object to change its speed, shape, or direction. Leaning on a desk applies a push force on the desk. This force exists even if the desk does not move (it could cause change if the net force was unbalanced). Force is applied to an object, not transferred. Energy is a property of a system associated with the extent of movement of an object or the amount of heat within it. Energy changes from one form to another can be tracked. |
Only living things have energy. | Energy can be transferred between objects. Batteries have chemical energy, cars have kinetic/motion energy. |
Only objects in motion have energy. | Potential energy is the energy stored in a coiled spring, in a battery (chemical energy), or in an object that can fall. |
Loudness and pitch are the same thing. | Pitch is how high or low a note is, while loudness is how loud or soft the note is. |
Sound moves faster in the air than solids. | Sound needs particles to be transmitted. The closer together the particles (solid) the faster the sound will move. |
You can see and hear an object far away at the same time. | Light travels faster than sound. |
You can scream in space. | Sound requires a medium (with particles close enough to bump together) to be transmitted. This does not occur in space. |
Heat is a kind of substance. | Heat is a form of energy that can be transferred or transformed. |
Things expand when heated to make room for heat. | When objects are heated, the particles gain kinetic/movement energy. This faster movement means they take up more space and the object can expand. |
Heat travels like fluid through objects. | Heat travels by conduction, convection, or radiation. |
Objects in the same room can be at different temperatures (metals will be colder than wood). | Metals are good conductors of heat. Touching metal in a cold room will conduct the heat away from your body (making your hand and the metal feel cold). Wood does not conduct heat as well as metal. This keeps the heat in your hand (wood feels warmer than metal). |
Things wrapped in an insulator will warm up. | An insulator maintains the heat in an object. A heat source is needed for an object to ‘warm up’. |
The eyes produce light (cartoon-like) so we can see objects. | Light is a form of energy that comes from a source (not human eyes). |
We see an object when light shines on it. | We see objects when the light bounces off an object and reaches our eyes. |
White light is a colour. | The primary colours of light are green, blue, and red. When these are mixed, they produce white light. |
A prism adds colour to light. | The different wavelengths of visible light bend in different amounts as they pass through a prism. This spreads the colours/wavelengths and produces a rainbow. |
The grass is green because it produces green light. | The green leaves of grass reflect most of the green light (and absorb other colours). This is why we see the green colour. |
The primary colours of light are the same as paint. | The primary colours of light are green, blue, and red. Mixing red and green light produces yellow light. |
When light moves through a coloured filter, the filter adds the colour to the light. | A coloured filter selectively absorbs some colours and lets others through. For example, a red filter only lets red light through. |
Light from a bulb only extends out a certain distance and then stops. | Light is spread over a greater area as it moves away from the source. This means there is less that reaches our eyes. |
A mirror reverses everything. | Mirrors reverse images left to right (not up and down). |
Light does not reflect from dull surfaces. | We see an object because it is a light source or the light has been reflected from its surface. |
Light from a bright light travels further than light from a dim light. | The brightness of a light indicates the number of photons (packets of light) that are able to travel from the source to your eye. The light always travels the same distance but spreads out. More photons will reach your eye even when far away. |
Static electricity and current electricity are two different things. | Both static and current involve electrically charged particles. In static electricity, the charged particles do not have a pathway to move. Current electricity has a pathway (usually wire). |
Energy in an electric circuit is used by a light globe. | Electrical energy is transformed at the globe into light and heat energy. |
Batteries store electrical energy. | Batteries store chemicals (chemical energy) that react to produce electricity in connecting wires. |
You only need 1 wire to make a circuit. | Electricity must be able to flow from the negative terminal of a battery to the positive terminal. If only 1 wire is used, the bulb terminal must be in direct contact with the battery terminal. |
The plastic in the wires keeps the electricity in (like water in a pipe). | Wires do not need plastic coating for the electrical current to flow. It does prevent potential short circuits. |
Current is ‘used up’ in a circuit. | The current stays the same value in all locations of a series circuit. |
The current moves from one end of the battery to the light. | When the battery is connected to a circuit, the current starts flowing in all parts of the circuit at the same time. |